Words by Jack Roper
By 2040, the zero-emissions aircraft market is forecast to grow to US$192 billion by investment bank UBS in the next 25 years. Already, the accelerated development of more electric aircraft is driving an urgent need for power-dense, airworthy, high-power electric motors.
“Demand is rising exponentially,” says Dr Hitendra Hirani, who manages the University of Nottingham’s Drives Specialist Services unit, which industrializes electrical motors and drives. “The end-solution may involve hydrogen, batteries or sustainable aviation fuels, but motors are critical to making any proposed drivetrain work.”
Electric motors in aerospace are not new. The Airbus A380 was the first commercial aircraft to apply them across multiple onboard systems and had four variable frequency electrical generators each delivering 150kVA. Today the Boeing 787 is one of the most electric airliners, with 1.5MW of onboard power and a bleedless environmental control architecture – both provided by Collins Aerospace.
The most novel aspect of increasing the use of motors in aerospace applications lies in evolving them into a primary propulsive power source.
“We’re leveraging 25 years’ learning from commercial and military aircraft to scale towards megawatt-type propulsion systems,” says Collins Aerospace senior engineering director, Marc Holme. “We are using electromagnetic design tools to optimize topologies and looking at new materials, cooling mechanisms and winding techniques.”
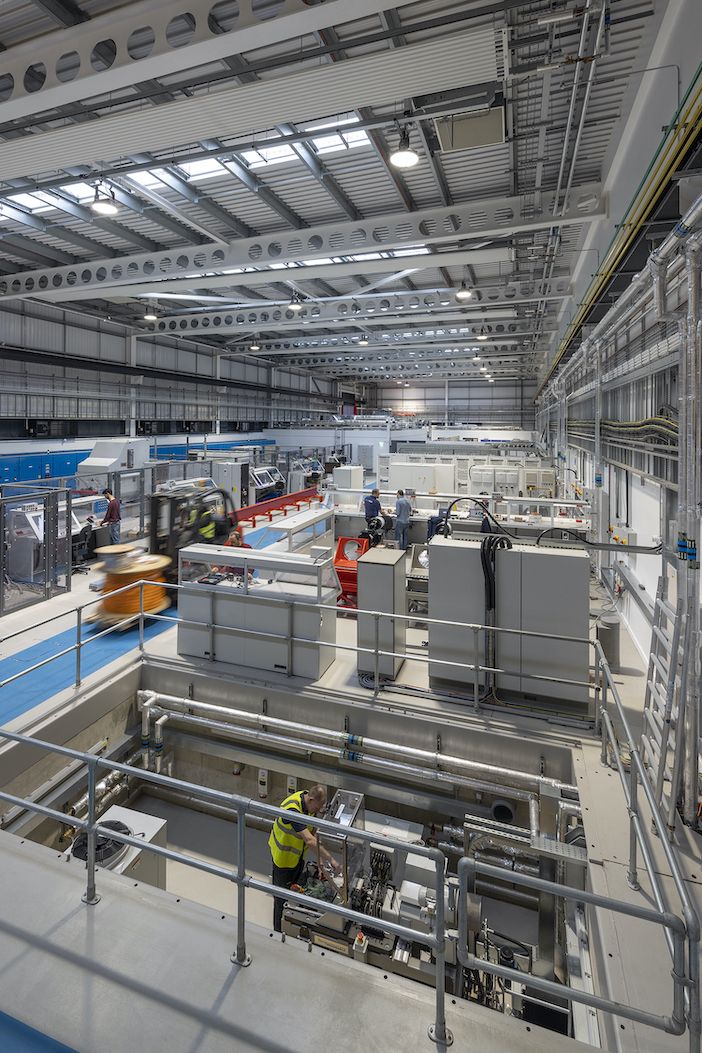
Requirements
Motor performance requirements vary between aircraft configurations and missions. For example, once borne aloft by helium, the Airlander 10 airship will need steady electric propulsion for forward flight. An urban eVTOL aircraft will need to regularly power take-offs. “The problems are similar, the solutions can be different,” says Hirani. “Mission profiles determine motor design, because rapid temperature- and power-cycling really accelerates degradation.”
In 2015 Airbus successfully flew the fully-electric E-Fan aircraft across the English Channel unaided. Seeing the merits and limitations of electric propulsion, four E-Fan engineers subsequently founded Ascendance Flight Technologies to develop the Sterna hybrid-electric powertrain and Atea, a winged VTOL with 400km (250 miles) range, 200km/h (125mph) top speed and an aspiration to fly at the Paris Olympics in 2024.
“Parallel architectures connect thermal and electrical engines to propellers with mechanical shafts,” says Ascendance’s hybrid director, Clément Dinel. “But Atea employs a series architecture which converts thermal power into electricity, distributes it by cable then converts electrical to mechanical power
for propellers.
“We make separate use of rotors for lift and propellers for horizontal push, avoiding the use of complex, hard-to-certify tilting mechanisms.”
The electric-thermal powertrain Sterna will support Atea and will be made available for us in other hybrid-electric concepts in VTOL and conventional aircraft.
“Today we use a turbogenerator,” says Dinel. “But Sterna is adaptable to other thermal energy-sources, aircraft sizes and motor power-ratings. The on-board calculator interfaces with every sub-component and dynamically allocates electric and thermal energy.”
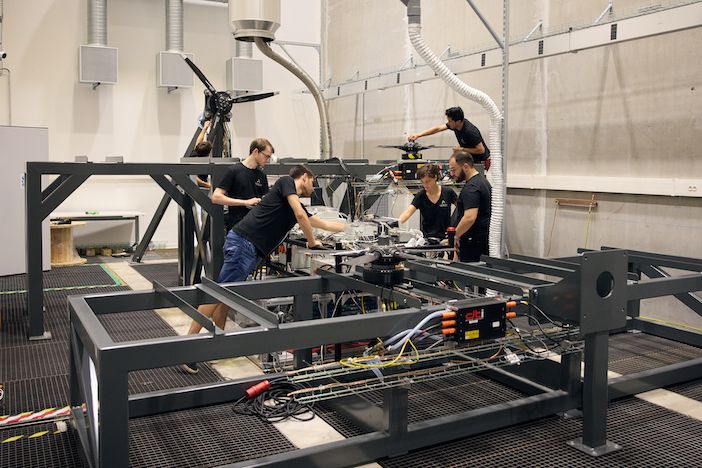
Having worked with manufacturers such as EMRAX, Ascendance considers power-density the principal driver of aerospace motor development, with permanent magnets, axial flux topologies and silicon carbide (SiC) inverters key enabling technologies.
Automotive to aerospace
A motors company making inroads into the aerospace market is Evolito. The company was spun out from YASA in 2021. UK company YASA successfully industrialized its acronymic yokeless and segmented armature for automotive applications during the last decade and Evolito is looking to build on that success in the aerospace sector.
“Creating prototypes is relatively easy,” says Evolito’s chief engineer, Mukesh Patel. “But you can lose 10% of performance scaling to production volumes. We bring YASA’s proven, high-volume industrialized capability from automotive into aerospace.”
Materials, magnets and electronics pioneered in automotive promise cost-reductions and robustness benefits. But not all topologies tailored to the road readily translate into aircraft. Electric vehicle (EV) motors may deliver 220kW, but a 90-seater aircraft could require around 8MW of power.
“The technology doesn’t necessarily scale in a linear way,” says Hirani. “A typical automotive motor scaled to 8MW would be too heavy to fly.
“Even if they were 99% efficient, 1% inefficiency at 8MW generates considerable heat to dissipate. That must be modelled and understood, but safety frameworks for testing megawatt aircraft systems don’t exist yet.”
“Motors developed in automotive are not necessarily acceptable for aerospace certification,” says Dinel.
“There are different norms for inverter control software. We have to mature suppliers to accompany us on the certification process.”
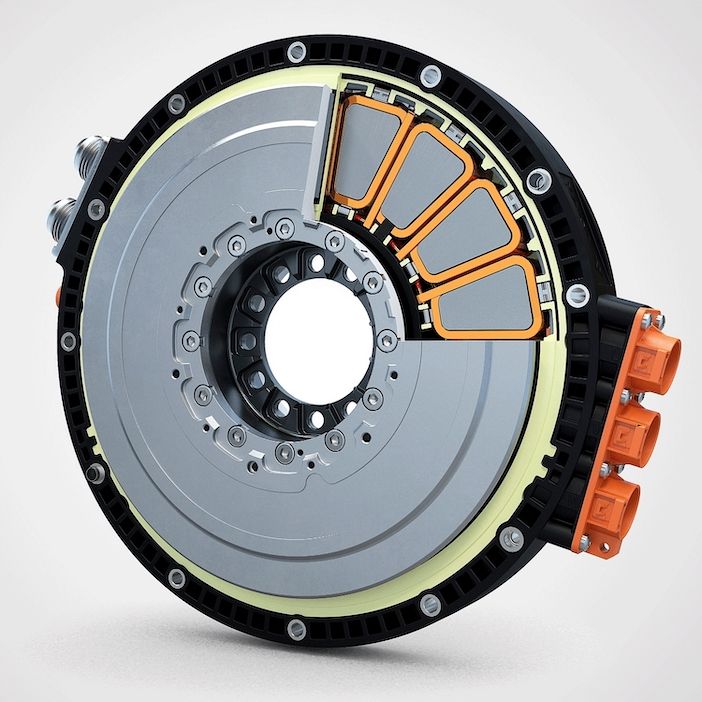
Temperature and pressure variations at altitude present acute motor integration challenges. Reduced air-density complicates heat dissipation and high-power systems are likely to require liquid cooling. Greater insulation and standoff capability is needed between high-voltage systems, since reduced pressure leads to partial discharge effects.
“Electricity behaves differently at altitude,” says Hirani. “The Corona Effect creates movements of charge not seen at ground level, which we must mitigate or prevent.
“We test for hundreds of hours to understand problems at the bounds of physics. If your EV breaks down, you can pull over and call a recovery service. But that’s not possible if motors fail in the air.”
Weight and volumetric constraints preclude making aircraft systems safe with additional boxing or insulation. Developers must innovate with dielectric coatings or potting, which fill high-voltage assemblies with gelatinous compounds to exclude gaseous effects. “On a wing, motors can be sewn back to -55°C,” says Holme. “Thermal cycling, partial discharge effects, vibration and moisture on components create a difficult mix for systems to survive.”
Degradation is the foremost cause of electrical failures. Over time, thermal cycling causes magnet performance to deteriorate and cables to fail. Nottingham University scientists are developing ways to prevent such problems.
“What if we could have coils without any insulation?” asks Hirani. “We’ve demonstrated that at 300kW and are scaling into higher powers. New magnetic materials could improve power-retention.
“Pre-configured windings could enable precise, automated insertion and eliminate the manufacturing variants of winding by hand.”
Test facilities and equipment
Fundamental to motor tests, a dynamometer is a test-stand equipped with a controllable electric motor, to which test motors are connected and driven under load from the dynamometer motor while torque transducers measure its performance.
“We run through our operating-range, measuring speed, torque and losses,” says Holme. “If it is
liquid-cooled we measure temperature-rises in the coolant. We look at the quality of waveforms produced. We will use dynamometers for both development and formal qualification.”
Evolito has invested in test facilities to accelerate learning in-house. These encompass environmental, electromagnetic and thermal testing facilities, bespoke high- and low-speed dynamometers and regeneration technology to reduce demand on the local grid.
“We’ve looked beyond today’s requirements to anticipate the industry’s future needs,” says Patel. “That’s quite responsible in an emerging market.
“We have devised accelerated life-testing methods to shake down the equipment before it gets anywhere near an aircraft and avoid problems coming back to our doorstep.”
Ascendance will conduct functional and performance testing in-house, then use other European facilities for specialized qualification tests. Investment in a full-integration test-bench allows it to test assembled powertrain components together.
“We’ve seen how real life differs from data sheets,” says Dinel. “On the test bench we can put the whole system through its flight cycle, understand interactions not evident from component tests and address thermal integration and heat dissipation.”
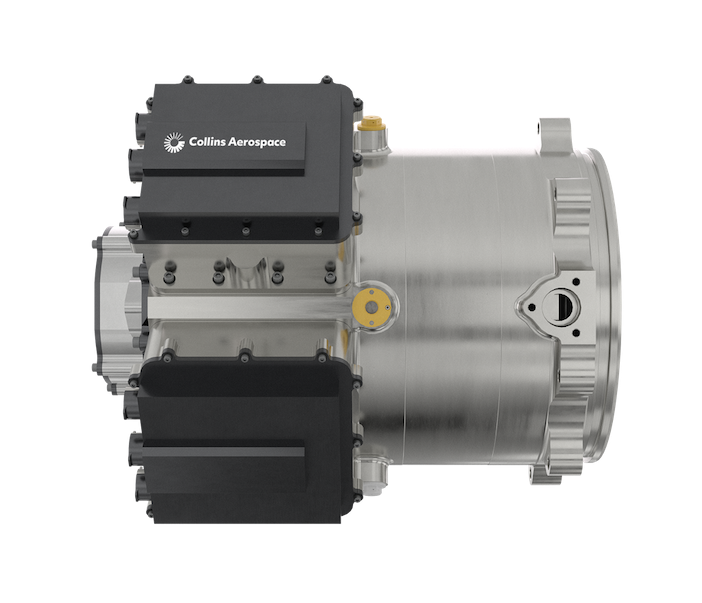
1MW motor
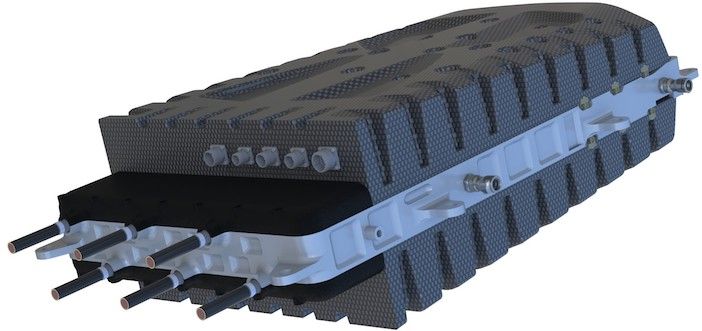
Collins Aerospace recently developed and tested a 500kW motor for the Hybrid Air Vehicles (HAV) Airlander and a 1MW motor which fully integrates with a Pratt & Whitney engine. The resulting 1MW powertrain will propel a Raytheon Technologies Dash 8 regional hybrid-electric flight demonstrator.
The hybrid-electric engine successfully completed its first engine run in December 2022 and is scheduled to fly next year.
“The demonstrator’s target is a 30% fuel-saving,” Holme says. “Using electrical energy in the climb phase allows for optimization of the thermal engine during cruise flight.
“As with commercial aircraft, we will run tests to demonstrate our system meets flight requirements. There are suites of environmental tests and combined powertrain tests covering all aircraft operating-points.”
The Grid, now under construction at Collins’ plant in Rockford, Illinois, is a US$50m, multi dynamometer electrical systems laboratory capable of emulating entire hybrid-electric aircraft and flight cycles. The Grid’s installed capacity can exceed Nottingham University’s 22MW capacity (See box: Motor testing for hire) and at these power levels, safety is paramount.
“In mechanical setups, you see an engine running and don’t put your finger in it,” says Hirani. “But in electrical environments, you don’t see the danger.
“We implement safety at rig, laboratory and building levels. Our high-power laboratory has restricted access, so nobody enters without completing an intense safety course.”
“We segregate operators from our test-bench in separate ambulatory and operating areas,” says Dinel. “Insulation of bare parts reduces electrocution risks. We have a clean, well-known earthing scheme and visual cues show whether the bench is powered.”
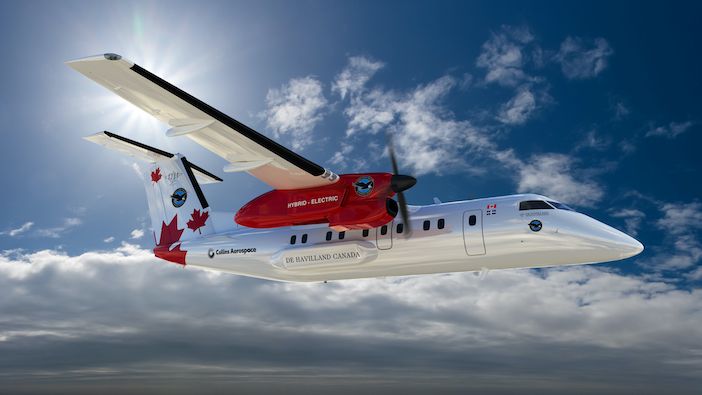
As Paris 2024 draws closer, it begins to look a challenging target for Atea’s first flight, while certification lies yet farther off. When Atea and Raytheon’s Dash-8 do fly, their power-systems will have been de-risked using ground-tests. Flight-testing will focus on non-regression testing and design-rule validation.
Fundamentally, Holme and Dinel see no insurmountable barriers to entry-into-service for full or partly electrically-propelled aircraft. However, the power density of batteries must improve further, while the main hurdle is an absence of certification frameworks.
“We can quickly demonstrate function and capability,” says Holme, “But developing certification requirements takes time. We need those requirements, especially around system safety and how we manage faults, to drive the architectures we can finally certify.”