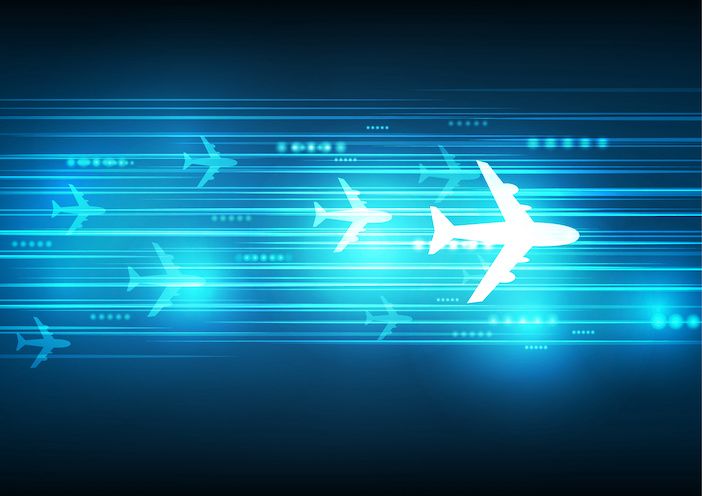
by Dr Suresh Perinpanayagam, senior lecturer in intelligent systems, Cranfield University,
The shift to electric and more-electric aircraft (MEA) is dependent on a vital technological factor other than strategy and investment. This pertains to what is termed as ‘reliable power electronics’ – a mesh of cutting-edge semiconductor devices and converter modules, the actual basis and enabler of an electrification revolution.
Power electronics bring with them some inadvertent challenges for aviation in terms of the optimal implementation and the need to build confidence amongst aviation technologists and the overall industry in their use.
Some shortcomings and challenges
There is undoubtedly a critical need for a standardized and reliable testing environment for OEMs and airlines themselves to rely on. This is because Prognostics and Health Management (PHM) of power electronics, both at the device and systems’ level, fall short of techniques and methods that could provide efficacious through-life management and optimal predictive maintenance.
Mission profiles of the critical applications of power electronics are not represented truly in qualification and laboratory tests. There are several stringent power device post-manufacturing reliability and qualification tests, such as electrical, environmental, and mechanical stress tests that are undertaken by manufacturers to assure their field-time reliability. But these are mostly only carried out under single environmental conditions, with test set-ups, rigs, and systems designed accordingly.
With the increasingly inherent integration of power electronics in MEA, especially power distribution, propulsion, and actuation systems, there is no room for compromise on the operational as well as functional performance of switches and discrete components that form vital power control and convert topologies.
Unknown failure mechanisms
There are significant challenges to overcome, including the issue of growing functional complexity, the increased population of components and intricate control algorithms that include existing electronic systems and software architectures.
New applications in aviation, typically operating under severe environments and extended hours, tend to pose cost constraints and more stringent reliability requirements besides ensuring safety compliance. Similarly, in this industry context, unknown failure mechanisms and thermal issues can be exaggerated with the continual increase of power density in converters and enhanced integration of power electronic systems.
There are uncertainties relating to the reliability performance of new Wide-Band Gap (WBG) materials and packaging technologies, including SiC (Silicon Carbide), GaN (Gallium Nitride), and HEMTs (High Electron Mobility Transistors).
The prevalent business landscape also poses a challenge. Shorter time to market cycles and soaring financial pressures are resulting in time and cost constraints for reliability assessments and the validation of the robustness of power devices.
A way forward
What’s needed is a highly-representative test environment. One that is nearly an exact replication of a real manufacturing/field environment, of physical and electrical operating conditions as well as the hardware and software configurations involved. Only in this way will it be possible to have a reliable platform for identifying defects and providing the kinds of cycles of improvement necessary for electrification to prove its potential.
PERTS
Researchers at Cranfield’s IVHM (Integrated Vehicle Health Management) Centre are working on PERTS (Power Electronics Reliability Testing System) as a solution to the problem, as well as expanding understanding of the physics of failure associated with state-of-the-art power semiconductor devices in our new world of electrification.
Once fully developed and tested, PERTS will enable optimized reliability testing and robustness evaluation of silicon-carbide and gallium nitride-based switching devices and modules, both independently and in PCB configuration. It will provide an effective set-up for combined power, temperature, and vibration cycling as well as stressing, including thermal and mechanical shock testing.
Predicting failures
A life-time failure prediction simulation (LFP) approach, developed by Cranfield researchers, is being used as a reinforcement, to make fast and accurate life predictions for power electronics at the system, board and component level in early design stages.
The capability to accurately model silicon-metal layers, semiconductor packaging, printed circuit boards (PCBs) and assemblies allows the prediction of solder fatigue failures due to thermal, mechanical and manufacturing conditions. The technique also allows a life cycle prognosis of the board to be made in terms of the environmental stresses it will encounter during the period of operation of more-electric landing gear system, for instance.
The LFP process, as shown, begins with the identification of the potential stressors which lead to failure, such as thermal events and cycling), mechanical shock and vibration, chemical, electrical, physical or any structural stressors that are liable to cause failure within the design. The power device / module / system is then exposed to highly accelerated stresses, which may be in terms of a finite element analysis FEA for thermal and mechanical loads, and the dominant cause(s) of failure is (are) determined.
By using models of the dominant failure mechanisms (of how and why the failure takes place), the failure data is generated – which can be subsequently processed to estimate lifetime and make health predictions inter alia prognostics.
Optimised PHM
A highly accurate prognostics and health management (PHM) regime is essential: enhanced real-time monitoring of dynamic electrical and physical parameters, such as on-state voltage (collector-emitter), resistance (drain to source), current (collector-emitter), and junction temperature under mission-profile conditions.
Following on from this, to produce an accurate assessment of stress that the device may endure during its lifetime, the mission profile needs to be considered as closely as possible.
For instance, a power electronics unit for electrical braking on an aircraft may see little usage during the flight. After take-off, being situated near the landing gear, it is subjected to extreme temperatures (more than -20°C) during flight. The device is then relied upon to undergo a power-up, enduring a high start-up transient current and full operational temperatures approaching 100°C as well as being subjected to excessive vibration for a short period of time whilst the aircraft lands.
Confidence in technology
The major business and operational changes required for electrification will all be founded on confidence in the technology – and that won’t happen without rock-solid testing and new understanding of the complex challenges presented by power electronics. The right testing and early design regimes will mean improvements in safety, flight hours and landings, component removals and failures, no fault found, as well as the small matter of securing the future sustainability of air travel.
Dr. Suresh Perinpanayagam is senior lecturer in intelligent systems at Cranfield University and a chartered engineer at the Integrated Vehicle Health Management Centre, Cranfield University. Suresh’s expertise is in data-centric engineering and digital twins for intelligent systems. Suresh has a Bachelor and Master in Aeronautical Engineering from Imperial College London. He pursued his PhD at the Rolls-Royce University Technology Centre at Imperial College, under the auspices of the European FP7 programme. During this tenure, he worked with major engine manufacturers in Europe (Rolls-Royce, UK; MTU, Germany; SNECMA, France).