While the number of hypersonic weapons and aircraft development program is increasing, engineers have to innovate so testing can enable the move into operational systems such as aircraft and weapons
Flying faster than Mach 5 is an old idea. During the 1950s and 1960s US hypersonic flight research programs such as the X-15 rocket plane were the first to test the concept. Intercontinental Ballistic Missiles, which re-enter the atmosphere at hypersonic speeds were also developed around the same time.
During the decades since then several hypersonic vehicles have been developed for research, then shelved by engineers. But in the last five years there has been a resurgence in interest in flying faster than 3,000mph (4,800km/h) primarily in the USA, Russia and China.
For the first time commercial companies, such as UK-based Reaction Engines have made solid progress developing air-breathing engines. The hope is that in the future these engines will enable “spaceplanes” to fly through the stratosphere and above at hypersonic speeds. However, most actual testing activity for hypersonic flight remains focused on single-use weapons systems.
There are at least 18 military hypersonic projects around the world. It is estimated by market analysts that between 2015 and 2024, the US Government will spend almost US$15 billion developing hypersonic technologies and weapons systems.
Ground testing
There are two main types of hypersonic weapon systems currently. Hypersonic glide vehicles (HGV) are launched from a rocket, probably an existing ICBM, before gliding down to a target. Hypersonic cruise missiles are powered by high-speed, air-breathing engines, also known as scramjets, after they acquire a target.
The first testing phase for both types of system is extensive ground testing. There are very few facilities capable of testing hypersonic concepts and technologies. Northrup Grumman’s Aerothermal Research and Testing facility in Ronkonkoma, New York, is one of them.
Dan Cresci is chief engineer in charge of test services at that facility and has worked for Northrup Grumman in the area of supersonics and hypersonics for 35 years.
Engineers like Cresci have been dealing with hypersonic testing for decades in the form of rocket and rocket assist-powered propulsion experiments. According to Cresci, interest and research into hypersonics and airbreathing propulsion has “ebbed and flowed” in the years since then. But, the many programs have all had one thing in common – they have been firmly in the R&D phase and focused on propulsion.
“The difference is now we’re closer to actually developing these technologies into operational systems – weapons, space vehicles and manned aircraft,” Cresci says. “In the testing world that means we are doing longer duration tests, at higher speeds and testing a variety of technologies together in a single test.
“We’ve solved many of the technical challenges around propulsion and we’re moving on to test materials, controls and guidance systems. We have six different cells, each with capabilities for focusing on a different aspect of hypersonic flight ground testing.”
Tests recreate as closely as possible the conditions that will be experienced during flight. The data from the testing is used to refine models that predict hypersonic flight performance.
Tests also differ in size, from running entire engines in cells to subjecting coupons of materials to extremely high temperatures and pressure gas flows from a nozzle. Tests generally run for between 30 and 120 seconds.
“Our objectives – one of everyone’s objectives – is to run tests for as long as possible, because the operational systems will have flight times of many minutes and more,” says Cresci.
For propulsion testing, engineers are measuring the same key parameters they were 20, 30 years ago: pressure, temperature, airflow, fuel flow and thrust. Materials testing usually focuses on thermal structural interactions, with engineers looking for and measuring deformation patterns if the materials are ablative.
Simulating these hypersonic test environments requires high-temperature combustion of hydrogen, oxygen and air from a complex system of plant controls and massive sets of storage tanks. To simulate high altitude flight, the Ronkonkoma site also has a large vacuum sphere, similar to the chambers used to test space vehicles. A recently added ejector system has increased the vacuum sphere’s run time.
One of the fundamental changes to testing to happen during Cresci’s career is that the amount of data generated has increased substantially. “Over the years the density of measurements has increased probably ten times,” says Cresci. “Accuracy has increased significantly and we can compare pre-test CFD [Computational Fluid Dynamics] predictions much quicker.
“It means the iterative process of development can move a lot faster – we understand things like flow fields and engine response in the hypersonic domain much better.
“We always want to have a higher fidelity test, so investment focuses on upgrading instrumentation and data acquisition networks to obtain higher rates of data. It’s all about getting to flight. Ground testing always generates more data than flight testing, where costs and planning increase a lot.”
Flight time
Paul Cook is director of missile systems at Curtiss-Wright Defense Solutions (CWDS), a major supplier of instrumentation and data acquisition solutions to hypersonic projects.
Flight testing for weapons systems, including hypersonic ones, is done in phases – an early short-range flight to test the vehicle, a longer-range flight to test the propulsion, then an even longer flight to test propulsion and guidance.
A B-52 carries a prototype of the hypersonic-capable AGM-183A Air-Launched Rapid Response Weapon, during its first captive carry flight on June 12, 2019 (Image: US Air Force)
Cook says, “Many hypersonic missile programs are in the very early phase now, so they are using our instrumentation to measure parameters such as internal temperatures, pressures and acceleration. The later guided flights gather much more data that is normally telemetered.”
“The technology for hypersonic flight has changed but the instrumentation used for testing has not changed much. What is changing is being able to transmit data during flight.”
“Test engineers on the commercial side like to record their data, while on the military side they like to transmit it for safety reasons. “On the commercial side they want the vehicle to return, on the military side, the vehicle generally does not return.”
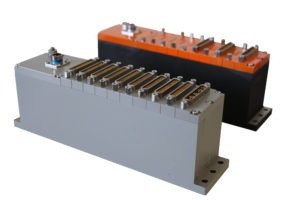
Instrumentation supplied by CWDS is off-the-shelf but is also highly configurable. A system designer can choose from thousands of different digital and analog signal conditioning modules that can be reconfigured to measure specific test points. “There’s not one set of measurements that all our customers want, so the modules have to support their different needs,” says Cook.
CWDS can supply the entire integrated system, including recorders, the instrumentation packages, guidance data interface, transmitters and tracking systems. According to Cook customers are increasingly taking up this option of an entire system to leverage expertise at CWDS.
Robustness
The FTI used in hypersonic flight testing has to be more robust than that used in conventional testing. 20 years ago, early hypersonic test vehicles would be made from material that would ablate away. “A vehicle would fly at a relatively low altitude at a very high speed, so the
aero-heating was significant,” says Cook.
“Today aero-heating is less of a concern because of advances in materials, which enable the latest hypersonic air vehicles to achieve faster speeds and longer distances.”
However, heat is still a concern within the missiles. Instrumentation and data acquisition packages must be as small and light as possible when integrated into flight test vehicles. “Thanks to integrated circuits and advances in packaging our instrumentation systems are 80% smaller than they were 20 years ago,” says Cook.
“But there are heat dissipation challenges that go with that, especially when something is operating at a very high data rate. You have to possess expertise in thermal management to solve those problems.”
Further compounding the heat challenge is that the amount of data being handled is expected to keep growing. “The data explosion is going to be uncontrollable. We’re working on 40GB/S links instead of the 20MB/S. The demand for data is so high that we will have to develop the technology to be very high speed. We will only be able to transmit at higher frequencies so we can transmit the data at a the wider bandwidths. It is a very exciting time.”
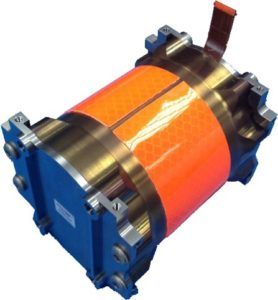
All of CWDS’ hypersonic FTI customers are doing short test flights, while studying the problem of how to collect the data from longer flights. Potential solutions include dedicated 5G links and laser-based satellite communications that link to the launch area. “The challenge is that the higher data rates will require higher rate infrastructure,” says Cook.
“Contractors would rent time on those networks to gather their data. To give you an idea of the data rates – we size our systems to use 100GB interfaces to record the data they produce today.”
The missile market is growing quickly for CWDS, not just in hypersonics, but across all platforms as engineers redesign systems to reduce size, keep costs down and fly further. But there is also a lot of testing happening in space launch systems.
Cook says, “The commercial launch industry is ramping up just as fast as Hypersonics to support launches for high data rate satellites and to resupply the space stations – that market is also exploding. We are working with six launch companies at the moment,” he says.
“But the missile market will remain our predominant product during the next decade over flight test.”
Hypersonic flight may be an old idea, but it has new ambitions – and test facilities, instrumentation and data will play a major role in achieving them.
The Talon hypersonic testbed
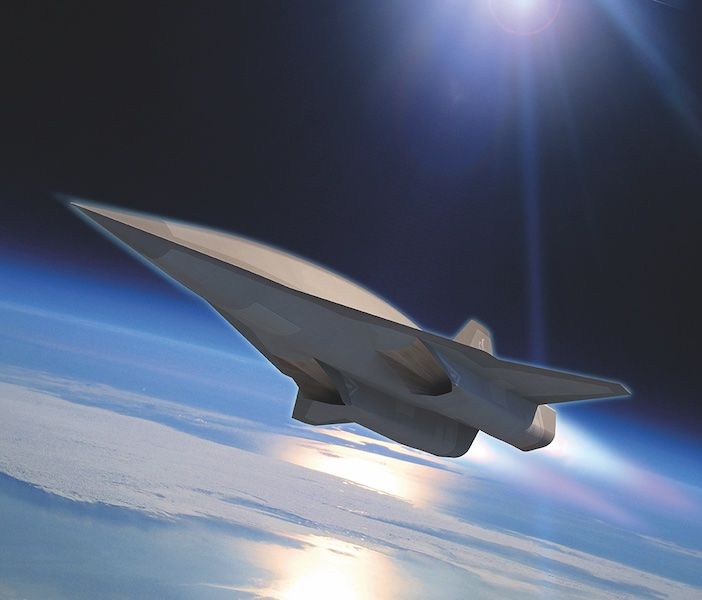
California-based company Stratolaunch is developing testbeds to provide easier and cheaper access to hypersonic flight testing, the first of which is Talon-A.
The Talon vehicles will launch from the Stratolaunch carrier aircraft, which has a wingspan of 385ft (117m) and was originally developed as an aerial launch platform for satellites.
Talon-A is a reusable, autonomous, liquid rocket-powered Mach 6-capable hypersonic vehicle with a length of 28ft (8.5m), a wingspan of 14ft (4.3m) and a launch weight of approximately 6,500 lbs (2,948kg). The vehicle has been designed to provide more than 60 seconds of hypersonic flight test conditions and then glide back for an autonomous landing on a conventional runway.
Talon-A is planned to be ready for use next year and will be able to fly 12 missions a year.
Rocket sleds for testing hypersonic missiles
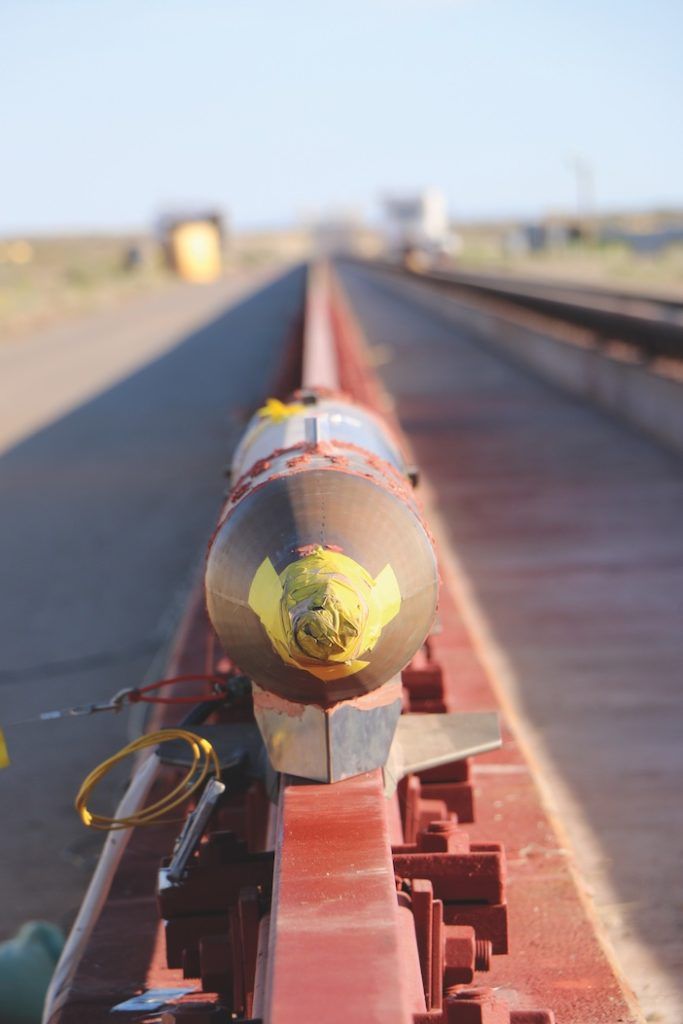
Holloman Air Force Base in New Mexico, USA hosts a unique facility, the longest hypersonic sled test track in the world.
Operated by the US Air Force’s 846th Test Squadron, the Holloman High Speed Test Track (HHSTT) is an almost 10 mile (16km) long monorail. During a test engineers fire sleds fitted with instrumented test articles along the straight and level track using repeated rocket firings at speeds of up to 6,400mph (10,300km/h). Portions of the track are fitted with sprinkler systems to simulate rain.
The sled testing provides a link between laboratory investigations and full-scale flight testing by simulating selected portions of the flight environment. The HHSTT was upgraded last year to prepare it for increased use during the next decade with a new braking system, a fiber-optic control and data system and new sled design.
Final testing of the HHST’s new systems and sleds is planned to take place this summer, starting with weather effects testing, such as simulated rain encounters. Testing of the US Air Force’s Air-Launched Rapid Response Weapon (ARRW) is expected to get underway next year.
Hypersonic propulsion systems being developed
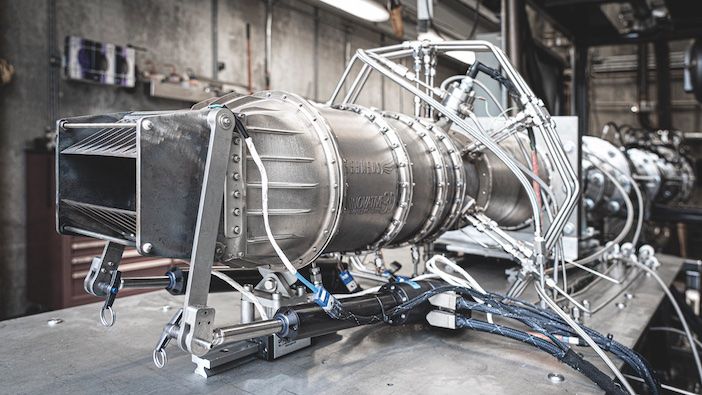
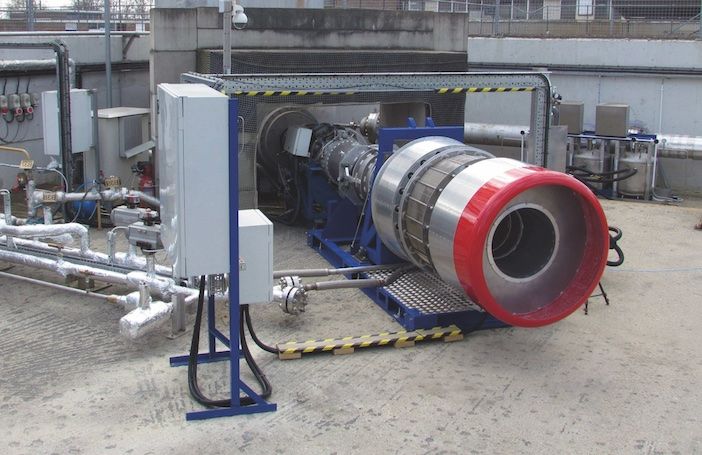
All existing hypersonic systems use liquid or solid rockets as their propulsion system and carry both fuel and oxidiser.
Reusable hypersonic air-breathing rocket engines have been researched for around 50 years but an operational system is yet to be deployed in the military or commercially. An air-breathing rocket engine gets the oxygen it needs to combust fuel from the atmosphere instead of from an oxidiser. This opens up opportunities for more efficient operations and the use of hydrocarbon fuels.
There are four companies developing air-breathing rocket engines for hypersonic air transport and travel. US-based startup Hermeus is testing a modified GE J85 engine. The engine will form the core of the turbine-based combined cycle propulsion system that will power its proposed 20-seat, Mach 5-capable passenger aircraft
Pratt & Whitney recently confirmed it is developing a hypersonic engine called the Metacomet based on the J58 engine that powered the SR-71 Blackbird spy plane to a record speed of around Mach 3.4 in the 1970s.
Meanwhile Reaction Engines is testing key parts of its SABRE (synergetic air-breathing rocket engine) ahead of a first fully-integrated engine test. The pre-cooler, heat exchanger and hydrogen have been validated so far.
Finally, Australia-based Hypersonix has conducted wind tunnel testing and plans to start hardware-in-the-loop testing of its Spartan scramjet propulsion system next year. The Mach 12-capable engine will be used in a spaceplane to deliver satellites to orbit.
Related stories
US Air Force detonates AARW hypersonic missile warhead
BAE Systems Australia increases funding for hypersonic missile development
Hypersonic startup raises US$16 million after completing engine tests
Rolls-Royce increases involvement in hypersonic air-breathing engine development project