by Jack Roper
While single-aisle aircraft produce half of all commercial aviation emissions today, Boeing expects demand for single-aisle and narrow-body airliners to increase by 30,000 in the next 20 years. Airbus and Boeing both believe increasing wing aspect ratios could improve their fuel efficiency by up to 10%, since lift generated across a longer, slenderer span creates smaller tip vortices and thus induces less drag.
“Lifting wings produce a large-scale motion of air,” explains UK Aerospace Technology Institute (ATI) deputy head of whole aircraft technology, Stuart Gates. “One result is a drag force which acts to oppose propulsion system thrust and depends on wing-lift distribution. Longer-span wings generally reduce this drag, thereby reducing fuel consumption.”
Extending wings moves wing loads outwards from the fuselage and increases their susceptibility to turbulence. But adding weight to strengthen wings would counteract their intended efficiency benefit. The NASA-Boeing Sustainable Flight Demonstrator (SFD) proposes a structural solution – the Transonic Truss-Braced Wing (TTBW).
“A truss-braced configuration minimizes the weight penalty of longer wings,” explains NASA’s SFD project manager, Brent Cobleigh. “The strut allows us to go longer, lighter and thinner.
“The junctures between wing, strut and fuselage are challenging for flow separation and localized shockwaves, but computational fluid dynamics has helped shape the design.”
The concept germinated from Boeing’s Subsonic Ultragreen Aircraft Research (SUGAR) program, conducted between 2009 and 2021 in response to a NASA solicitation to identify future aircraft technologies. Consecutive SUGAR studies evolved the sweep of the wings and interrogated their structural stability.
“It’s easy to draw concepts and dream,” says NASA Sustainable Flight National Partnership (SFNP) mission integration manager, Richard Wahls. “But if the wing’s span, thinness and flexibility meant adding weight to stiffen it, we would lose the benefit. In 2013, a test in the Langley Transonic Dynamics Tunnel allowed us to structurally scale the aircraft’s geometry. We learned that, while we couldn’t ignore aeroelasticity, it wasn’t a show stopper.”
The program will modify a McDonnell Douglas MD-90. Boeing will leverage its in-house experience with the aircraft, which is comparable in size to current single-aisle aircraft. Its 108ft (33m) wingspan will be extended and relocated upwards in an experiment on a scale unseen in civil aviation since the 1950s.
“The MD-90 has low wings and two engines at the back,” says Wahls. “The low wing will be removed and the strut mounted in its place, to capitalize on existing structures.
“The new wing will be mounted on top, rear engines replaced with modern engines mounted under the wing and a forward fuselage section removed for balance.”
Active versus passive
But the SFD isn’t the only game in town. Airbus will flight-test high aspect ratio wings in its X-Wing project using a Cessna jet, less ambitious in scale but more immediate in timescale. Where Boeing has adopted a structural solution, Airbus will use active wing technologies – flapping wingtips, multifunctional trailing edges and pop-up spoilers – to dynamically mitigate the load susceptibility of longer wings. The UK Government-backed ATI is part-funding the project.
“Our Destination Zero strategy focuses on ultra-efficient and zero-carbon aircraft technologies and cross-cutting enablers,” says Gates. “Wings feature heavily throughout. The UK is a leader in wings and propulsion, areas poised for dramatic changes with associated competitive threat, so we must capitalize on our strengths.”
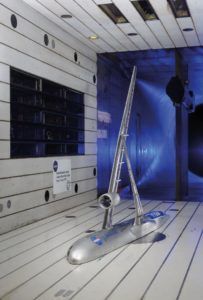
Gates believes intensive focus on sustainable aviation fuel (SAF) or hydrogen propulsion can obscure the ongoing importance of aerodynamics to whole-aircraft emissions reduction goals.
“It is a necessary and fuel-agnostic enabler for other technologies,” he says. “Investment in aerodynamics is a worthwhile means to reduce fuel burn, whether the ultimate route is SAF or hydrogen.”
The NASA-Boeing TTBW configuration will not initially incorporate active wing mechanisms, but NASA has tested conventional cantilever wings with active trailing-edge devices coupled to sensors. In 2013, it tested a truss-braced wing with active controls to study flutter-suppression and aeroelastic phenomena.
“We didn’t appear to need active wing technologies to make TTBW a reality,” says Cobleigh. “But all configurations evolve over time, so they could create incremental efficiency gains in the future. We always ask ‘does that technology buy itself onto the airplane? Is the cost of developing and implementing it worth the benefit?’ Eventually, manufacturers will make those decisions.”
Bend it until it breaks
NASA has the authority to certify research aircraft as safe to fly without recourse to the Federal Aviation Administration (FAA). A rigorous airworthiness process will include structural ground tests, the data from which will be matched to predictions to clear the demonstrator for flight.
“Loading the structure with jacks or weights will simulate loads it will feel in flight up to the edge of its envelope,” says Wahls. “In commercial programs, there’s an ultimate test where they load the airplane, barely visible for all the rigging, until it breaks. We won’t break ours, but go to a sufficient safety margin. Dynamics tests on a shaker will find its modes for comparison with predictions.”
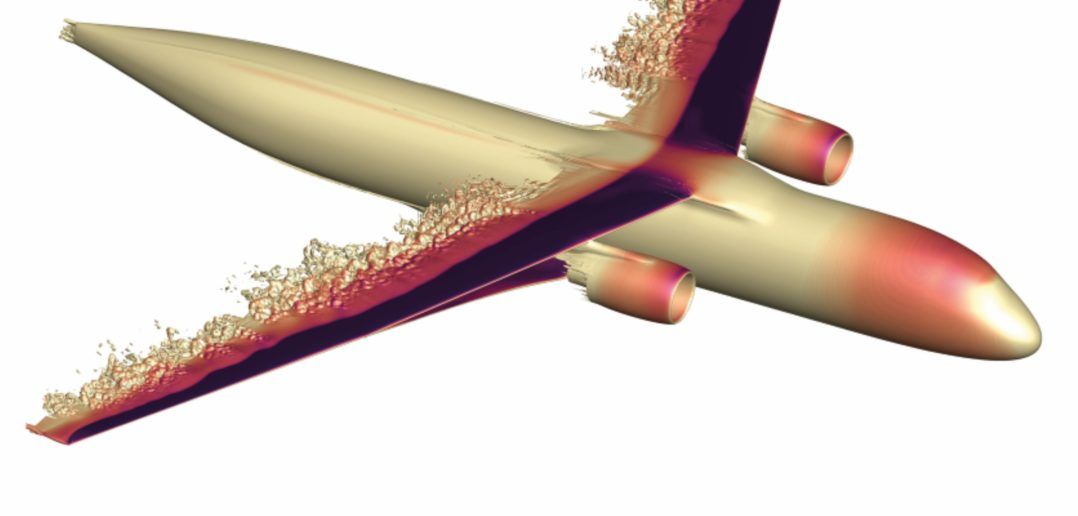
Once the vehicle is integrated, NASA will support a wing-bending test conducted by Boeing. NASA test facilities will continue playing a crucial role, with a high-speed test planned at the Ames Unitary Plan Wind Tunnel.
“That’ll use a large, 11-ft test section up to Mach 0.8,” says Cobleigh. “We will test a full-span model, then half of the airplane, sliced down the center. That allows us to double the size of wing we put in the tunnel and obtain higher Reynolds-number data. Our subsonic wind tunnel schedule is busy, so Boeing will use QinetiQ’s tunnel in Farnborough, UK to test low-speed aerodynamics.”
Airbus has tested a partly 3D-printed scale model of a jet with lightweight, long-span wings in its low-speed wind tunnel at Filton, UK. Together with computational aerodynamics analysis, this provides a valuable way to carry out pre-flight validation. Gates believes the demise of wind tunnel tests in favor of computer simulation may be exaggerated.
“Our stakeholders see physical testing as essential for understanding future aerodynamics,” he says. “It is about using the wind tunnel as part of that numerical simulation effort and ensuring the data is compatible.
“Pressure-sensitive paint allied to cameras can produce visualizations of surface pressures which resemble numerical simulation imagery, but from a physical test. Measuring model deformations under load will be important for evaluating future high-span flexible wings.”
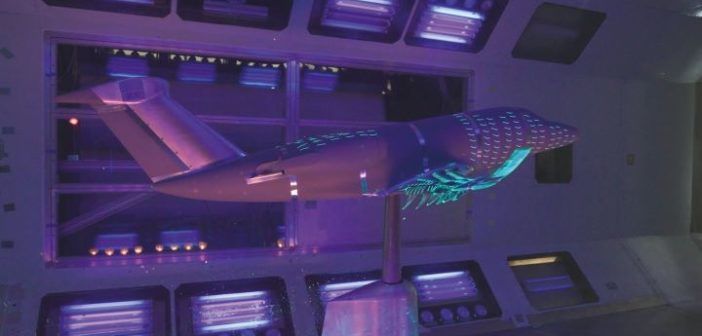
NASA will use piloted simulation to develop flight test maneuvers and emergency procedures. Despite its altered configuration, the SFD aspires to fly like a normal aircraft, providing familiarity for pilots and a smooth ride for passengers. Simulation will be vital to tailoring its behavior based on control laws.
“We will start off simple with the simulation, as we always do, then add complexity over time,” says Cobleigh. “We’ll look at control system anomalies and inject different failures with pilots in the loop to identify
non-recoverable failure conditions. We will check out software, assess loads during flight. Simulation is endlessly useful for validating systems.”
But some learnings can only be made during flight. Whilst the Langley and Ames tunnels allow realistic tests of structural characteristics and aerodynamics respectively, the two can be combined only by flying a structure of this scale in real conditions. The demonstrator should commence a one-year flight campaign in 2028, bedecked with strain gages, accelerometers and other sensors to measure aerodynamic performance.
Cobleigh says, “In flight we learn the unknown unknowns, the aspects we couldn’t quite predict but need to learn before launching a $10 billion development effort. How do the structure, truss and wing interact under real conditions? Are structural interfaces designed properly – or over-designed? That feedback is critical to the design loop and helping manufacturers predict aircraft performance.”
In Toulouse, Airbus will fit its Cessna with probes and antennae for uncrewed operation in remote-controlled flight tests. Scheduled to take place during fall 2024, the campaign aims to clock 100 flight hours from the French Air and Space Force Base at Cazaux, providing representative data to inform development of single-aisle aircraft wings.
The SFD will retain conventional turbines as NASA develops advanced propulsion and hybridization across separate SFNP projects to better manage risk. When nascent technologies do combine, the TTBW architecture may happily accommodate larger-diameter propulsion systems.
“Since the 1970s aircraft engines have grown larger and closer to the ground,” says Wahls. “This high wing takes us back up and gives engine designers more degrees of freedom.
“We are locked into the MD-90 fuselage, which we might have designed differently from scratch. Getting your desired learnings within a money and time budget does involve compromises.”
The 30% fuel efficiency improvement target potentially includes 10% from wings themselves, 10% from core engine efficiency, 5% from hybridization and savings from propulsors and lightweight composites. But complex trades in combining these innovations may preclude reaping any one’s full benefit.
“The challenge is whole-aircraft integration,” says Gates. “We may lose some of that 10% aerodynamic benefit due to integration penalties. ATI models predict that overall efficiency improvements of 16% for wide-body and 20% for single-aisle next-generation aircraft are possible. It’s about combining energy-efficient airframes with advanced propulsion.”
Edge-of-envelope studies on topics like ice-formation around novel TTBW junctures will run parallel to flight preparation. Beyond 2029, knotty integration trade-offs must be resolved and composite production rates increased to match those of today’s airliners, a challenge already occupying Airbus and Boeing. Aircraft may also need longer wings to fold when accessing airport infrastructure. As climatic storm clouds gather, aviation must work fast to keep pace.
Creating sustainable flight
Boeing’s Transonic Truss-Braced Wing (TTBW) design relies on supporting struts to safely increase wing aspect ratios without increasing their weight.
The NASA-Boeing Sustainable Flight Demonstrator (SFD) project aims to flight-test the TTBW on a modified MD-90 in 2028. It could contribute to up to a 30% increase in single-aisle airliner fuel-efficiency by the 2030s.
“These gains derive from the TTBW system and expected improvements in propulsion systems, materials and system architecture,” says Boeing chief technology officer, Todd Citron. “In 2028, the full-scale SFD demonstrator will take to the skies on ultra-thin wings, braced by trusses, with a longer span for higher aerodynamic efficiency. The configuration will also free up underwing space for advanced propulsion systems.”
NASA is committing US$425 million via a funded Space Act Agreement, while Boeing and its industry partners will meet remaining costs estimated at US$725m. NASA believes this public-private partnership will accelerate high-risk technology development crucial to 2050 net-zero targets.
“The key output will be integrated
aircraft-level learning, as opposed to individual technology piece-parts,” says Citron. “The results of this effort – and market conditions – will dictate whether this shows up in the next commercial product.”
NASA’s Sustainable Flight National Partnership encompasses parallel projects linked to the overall 30% target, including work on turbine engine efficiency, mild hybridization and increased production-rates for composite aircraft structures.
“This partnership was a decade in the making and now we find ourselves at a critical junction,” says Citron.
“Climate change and economic imperatives require bold action. SFD program technologies could shape future generations of single-aisle aircraft with breakthrough aerodynamics and drastically lower emissions.”
The active way to slender wings
Airbus aims to harness avian biomimicry to extend wing aspect ratios without adding to their weight.
The European aircraft manufacturer’s eXtra Performance Wing (X-Wing) project will fly a Cessna Citation VII business jet with 20m (65ft) composite wings equipped with active control technologies. These will allow the wings to adapt themselves to withstand gust loads despite their slender design.
The Cessna Citation’s wings will feature 2m (6.5ft) tip sections on semi-aeroelastic hinges. An onboard LiDAR system will anticipate turbulence one second ahead and activate a hinge drive-gear mechanism to release the wingtips, whose subsequent flapping will attenuate turbulence-induced wing-loads. Multifunctional trailing edges on each wing and wingtip pop-up spoilers will assist in dynamic load-mitigation.
“For any aircraft, the best fuel is the fuel you don’t need,” says Airbus head of research and technology, Dr Mark Bentall, speaking at the Sustainable Skies World Summit in Farnborough in April. “That means we need more efficient airframes. There’s a huge effort to develop high aspect ratio wing technology and achieve extreme levels of efficiency.”
Airbus’ previous AlbatrossONE project developed hinged wings which future airliners could use to adapt their wing-shape, enabling the use of high aspect wings without supporting structures or weight penalties.
“Wing-X has been wind tunnel tested and we expect a prototype to fly next year,” says Bentall. “Engines will be another contributor to improving efficiency. As an airframer, we’re most interested in engine integration. We’re also looking at industrialization, including new manufacturing technologies.”