Two years ago, Airbus unveiled several possible aircraft concepts – known collectively as “ZEROe” – which are helping to define the world’s first zero-emission commercial airliner which could enter service by 2035.
While these concepts explore various size categories, aerodynamic layouts and propulsion system architectures, they all have one thing in common: they are hydrogen-fuelled. Three of them have engines that use hydrogen combustion to drive their gas turbines – similar to the way that turbofans and turboprops burn kerosene today, but without the latter’s CO2 and particulate emissions.
Meanwhile, a fourth ZEROe concept aircraft, representing a high-wing 100-seat regional airliner, features six eight-bladed propellers attached to engine pods – a configuration recently patented by Airbus. While outwardly resembling turboprop powerplants, these pods actually contain hydrogen fuel cells that produce electricity as the result of an electrochemical reaction to power electric motors.
It is in this context that Airbus has been conducting feasibility studies and laboratory tests to realize a fully working megawatt-class fuel-cell engine and demonstrator which could be tested in flight by the middle of this decade – around 2026.
First announced publicly at the end of November at the Airbus Summit, the demonstrator will use Airbus’ multi-modal flight test platform – A380 MSN001. The aircraft will be modified externally to carry the fuel-cell engine pod, while inside the aircraft’s rear fuselage Airbus will install a unique cryogenic tank to contain the liquefied hydrogen.
How it works
For this proof-of-concept demonstrator, liquid hydrogen from the cryogenic tank is converted into a gaseous state. It is then distributed to the fuel cell via supply lines running from the tank and through an external stub aerodynamic and load-bearing support structure to the engine pylon interface.
From there the gaseous hydrogen enters the fuel cell inside the pod, where dioxygen (O2) molecules are also supplied by a controlled flow of air – taken from the surrounding atmosphere. The resulting reaction inside the fuel cell produces direct electrical current (DC) which is subsequently converted into alternating current (AC) by the means of inverters.
Electric motors near the front of the pod then convert the electrical power into mechanical power by communicating torque to a reduction gearbox. At the end of the propulsion chain the propeller provides the thrust.
The thermal energy generated by the fuel cell needs to be conveyed by a liquid cooling system to heat exchangers where it is dissipated into the ambient air. Water is also produced as a byproduct of the electrochemical reaction which is expelled from an outlet at the back of the pod.
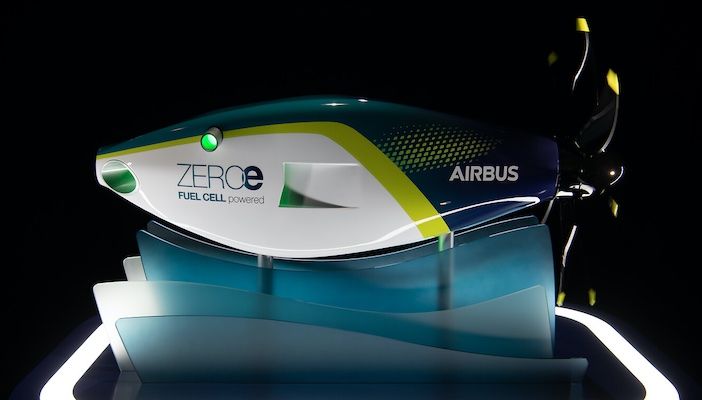
Modifying the A380
The A380 was the obvious choice as ‘host’ for the hydrogen fuel-cell engine demonstrator. “It has plenty of space internally – so there are no constraints in terms of accommodating everything we need, as well as the ability to test multiple configurations,” says Mathias Andriamisaina, head of ZEROe demonstrators and tests at Airbus.
“In terms of aerodynamics, the A380 is a very stable aircraft. So the pod attached to the rear fuselage via the stub doesn’t pose much of an issue. Furthermore, the airflows from the pod and its propeller do not affect the airflow over the A380’s tail surfaces.
“With the A380 we also have an aircraft that’s already fully instrumented. The flight-test-instrumentation is a big part of the project and can be a big driver in terms of cost and planning. So MSN001 was the perfect fit for us.”
The overall concept the teams settled on was to make the minimum amount of structural modification inside the A380. “We are just reinforcing two frames of the fuselage and then fitting this stub externally. Then we are fitting a specially adapted pylon to attach the structure of the pod onto this stub. Overall it will be able to support high sustained static and dynamic loads,” Andriamisaina says.
While most of the equipment is installed outside the aircraft, inside there is what the team refers to as the “tent” – a 10m (32ft) long, 4m (13ft) wide housing with a parabolic cross-section, which will be crafted out of carbon fiber by an Airbus Atlantic team at the Technocentre de Nantes, France. Inside this structure can be housed up to four cryogenic tanks containing liquid hydrogen.
During flight tests, the actual air inside the tent will be at the same pressure as outside the aircraft. In other words, the equipment installed inside this tent will only see the atmospheric unpressurized environment. This includes the hydrogen supply lines from the tank, which is routed directly from the tent to the stub and then to the externally mounted pod, which is to be assembled at Airbus’ factory in St Eloi in Toulouse.
“Using this approach avoids the risk of any hydrogen entering into the A380’s main pressurized interior because all the hydrogen routings from the tank will also be located inside our protective tent environment. There are also emergency venting lines, so we can expel the hydrogen out of this emergency line if needed,” says Andriamisaina.
Next steps
“Everything concerning the distribution center and the motor controller unit are being produced internally – this is an ‘Airbus’ activity,” says Andriamisaina. “In addition, the propelling system and the gearbox associated and the pitch control is developed by own Airbus Helicopters and Defence and Space colleagues. It’s the first time that Airbus has created such an engine ourselves – it’s a big challenge.”
Other firsts for Airbus’ include the adoption of a high-voltage distributed electrical architecture for up to 1,000 Volts DC and also the creation of a bespoke engine control system – this will translate the thrust commanded by the crew into the actual shaft power.
Extensive ground testing activities are already well underway. Examples include electrical benches, thermal management systems and several fuel-cell integration benches. From 2024 there will be additional integrated test benches such as: a fuel cell system integration bench featuring a 200kW fuel-cell stack and a climatic chamber; a functional integration bench for the engine control system to validate real interfaces and actuators; and a Propeller Integration Bench. The latter will explore propeller pitch actuation gearbox integration, gearbox endurance, and partial blade-release tests.
In parallel, the newly developed power centers and power converters for the fuel cell flight demonstrator will be connected to the A380 “iron bird” test rig so as to integrate them with the legacy A380 ATA Chapter 24 hardware (electrical power).
Later that year there will also be an “iron pod”. This test rig will bring together for the first time various key ingredients: 800kW of fuel cell power to two electric motors; the motor control units; the gearbox; the new high-voltage electrical distribution and conversion system; a ‘flight’ air supply and the liquid cooling system.
By 2026, and all being well following the various integration bench tests, the teams will be able to test the entire system in the integrated test bench (ITB). This will comprise the flight-standard fuel cell pod, complete with all its various systems and of course the propeller.
The ITB, which will be built in 2024, will be connected to the same flight test instrumentation as for the A380 MSN001 and there will also be a co-located control room for flight crew training.
“Between now and the flight tests in 2026 we have a lot of challenges ahead, not only in design for sure but also in the testing,” says Andriamisaina.
This article by Martin Fendt, Airbus can be found in its original form here.